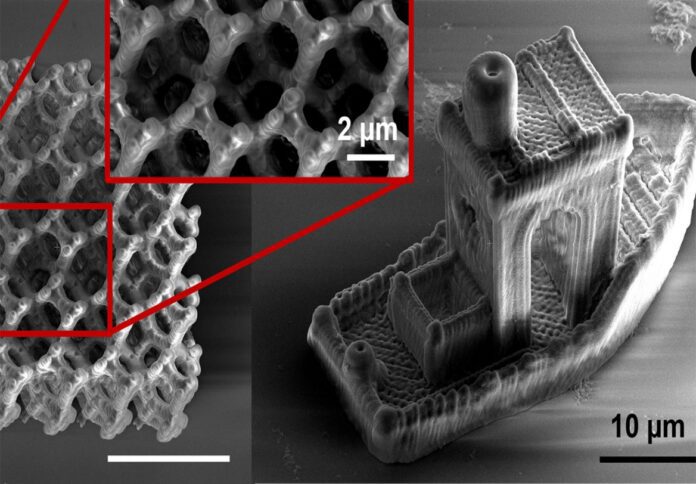
Researchers at the University of California, Berkeley, have developed a groundbreaking fabrication technique that allows the creation of complex 3D structures for quantum sensing particles.
These novel sensors can precisely detect changes in temperature and magnetic fields in microscopic environments, marking a significant step towards practical applications in materials science, biology, and chemistry, the university said in a news release.
The research findings, detailed in the journal Nano Letters, demonstrate the use of additive manufacturing methods to produce highly customisable 3D structures capable of hosting minuscule diamonds containing quantum sensing elements.
These 3D-printed quantum sensors offer the capability to make sensitive measurements at room temperature, potentially opening the door to transformative applications across various fields.
Co-lead author of the study and a graduate student in the Department of Mechanical Engineering, Brian Blankenship, highlighted the immense potential of this technology.
He stated, “In a couple of years, this technology might be used to incorporate sensors into microfluidics, electronics and biological systems — and open new avenues for the widespread utilization of quantum sensors for other applications that we haven’t even thought about yet.”
Costas Grigoropoulos, co-principal investigator and professor of mechanical engineering, emphasised the significance of this new fabrication technique, enabling tailored and precise design of structures with desired properties.
“These architected materials are optimised to provide a tailored mechanical response,” said Grigoropoulos.
He added, “They combine sensing as well as actuation functionalities for applications in structural materials, tissue engineering and opto-mechanical systems.”
According to the university, quantum sensors leverage the properties of atoms and light to measure minute changes in magnetic and electric fields, strain, and temperature.
Currently, they are employed in some of the world’s most accurate clocks, powering GPS systems. There is also growing interest in utilising these sensors in various fields, including neuroscience.
One of the key challenges in utilizing quantum sensors outside of controlled laboratory environments has been the need for extremely cold temperatures.
“Many platforms for quantum sensing require extremely cold temperatures — hundreds of degrees below freezing — in order to function correctly,” explained Grigoropoulos.
“In addition, these materials often need to be very clean and perfectly crystalline, which can prohibit their use in many practical applications.”
To overcome these challenges, the researchers turned to additive manufacturing techniques to structure quantum sensing particles, known as nitrogen vacancy centers, into 3D configurations.
These centres, found within diamonds, occur when a single carbon atom is replaced by a nitrogen atom, with an adjacent carbon atom being empty.
Notably, nitrogen vacancy centers exhibit robust performance at room temperature and retain their quantum properties even when in particle form.
“Our approach overcomes the challenges associated with structuring single-crystal substrates, and these nitrogen vacancy centers can work reliably at room temperature,” said Blankenship.
He added, “We demonstrated that by using a modified microscope, we could take precise measurements of temperature and magnetic field measurements inside of these structures.”
The research was conducted at the Laser Thermal Laboratory, a research group affiliated with the Department of Mechanical Engineering, and received funding from the National Science Foundation.
The laboratory’s primary goal for this project was to design and fabricate complex hybrid materials using multiphoton lithography.